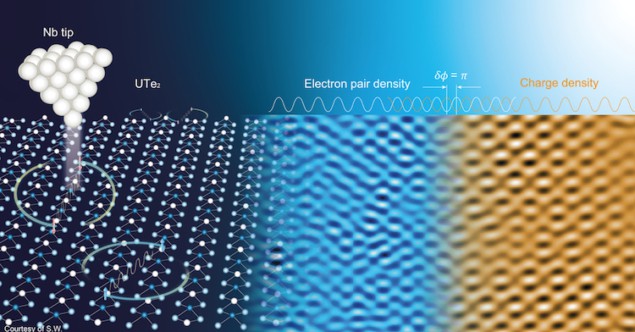
Researchers in the US, UK and Ireland have identified a new crystalline superconducting state in uranium ditelluride (UTe2). The existence of this state challenges the conventional picture of superconductivity and could have implications for the development of quantum computers.
â€ÅPhysicists have been searching for a material like this for more than 60 years,†says team leader Shuqiu Wang, a condensed-matter physicist and postdoctoral researcher at the University of Oxford, UK.
Superconductors are materials that conduct electricity without any resistance. In the Bardeen-Cooper-Schrieffer (BCS) theory of superconductivity, the transition to resistance-free current flow occurs at low temperatures, when electrons overcome their mutual electrical repulsion to form so-called â€ÅCooper pairs†that can travel unhindered through the material like a superfluid.
s-, p– and d-wave symmetries
The wavefunctions of these Cooper pairs have three possible types of symmetry: s-, d– or p-wave. s-wave superconductors include conventional (that is, BCS theory-obeying) superconductors such as lead, tin and mercury. In these materials, each Cooper pair consists of one electron with spin up and one electron with spin down. As these electrons move head-on towards each other, their net spin angular momentum is zero.
d-wave superconductivity occurs in unconventional (non-BCS) high-temperature superconductors such as copper oxides (cuprates). Electrons in these materials also form Cooper pairs where one electron is spin up and the other spin down, so the total spin angular momentum for each pair is again zero. However, the pairs’ orbital angular momentum is nonzero.
The final category, p-wave superconductors, is unique and highly distinct in that the Cooper pair wavefunctions of these materials have odd parity. This means that both electrons are either spin up or spin down, giving each pair a single quantum of spin angular momentum.
p-wave superconductors have attracted considerable attention recently because they are also topological superconductors, meaning that they harbour unusual states at their edges. These edge states, known as Majorana zero modes, may have applications in quantum computing. The search for technologically viable topological superconductors with odd-parity Cooper pairs is thus a hot topic in quantum matter research.
An electronic crystal embedded in the background superfluid
In the new work, researchers led by Wang and C Séamus Davis at Oxford, together with Qiangqiang Gu of Cornell University and Joseph P Carroll at University College Cork, used a technique called scanning Josephson tunnelling microscopy to visualize spatial modulations of the superconducting pairing potential in UTe2, a recently-discovered topological superconductor. This technique provides unparalleled microelectronvolt-scale energy resolution, allowing the electron pairing energy gap to be visualized at the atomic level.
The team’s observations revealed that some of the electron pairs in UTe2 form a crystalline structure within the background superfluid. Such structures are known as electron pair-density waves (PDW), and the team had previously observed them in s-wave superconductors and in d-wave superconductors. The latest results therefore demonstrate that the PDW state is common to all types of Cooper-pair symmetries.
Unconventional superconductor is even odder than expected
â€ÅWhat is exciting for us and the superconducting community at large is that the PDW we discovered appears in a bulk p-wave superconductor,†Wang says. She adds that the pairs of electrons appear to have intrinsic angular momentum: â€ÅIf this is true, then what we have detected is the first PDW composed of exotic spin-triplet pairs of electrons in which the spins of both electrons point in the same direction. While such states do exist in p-wave superfluid helium-3, they are unprecedented in superconductors.â€Â
A quantum bit material for topological quantum computers
UTe2 was first synthesized five years ago and there is growing evidence that it might be used to make quantum bits, or qubits, in so-called topological quantum computers. The edge states in such computers would be protected, and therefore robust to a phenomenon called decoherence in which qubits lose their quantum nature (and the information that is encoded in them) due to interactions with their environment.
â€ÅUTe2 could thus enable more stable and practical quantum computers,†Wang tells Physics World. â€ÅOur work is a crucial step towards understanding this intriguing material and unlocking such applications.â€Â
The team’s results are published in Nature.